Difference between revisions of "Lactobacillus"
m |
m |
||
Line 413: | Line 413: | ||
===Bacteriocins=== | ===Bacteriocins=== | ||
− | While most species of ''Lactobacillus'' do not produce bacteriocins, many strains of ''L. acidophilus'' are well known for being able to produce bacteriocins, including probiotics strains. Bacteriocins are similar to the [[Saccharomyces#Killer_Wine_Yeast|toxins that some wine yeast strains produce]], however bacteriocins tend to target other species that are closely related to the toxin-producing species (although there are some exceptions to this in the literature). The bacteriocin that ''L. acidophilus'' produces is a narrow spectrum class II bacteriocin, lactacin B (narrow spectrum means that this toxin kills a very narrow range of closely related Gram-positive bacteria). Species that are susceptible to the lactacin B toxin include species that are genetically closely related to ''L. acidophilus'': ''L. leichmanii'', ''L. bulgaricus'', ''L. delbruekii'', ''L. lactis'', and ''L. helveticus''. Species that are insensitive to the toxin are ''L. plantarum'', ''L. casei'', ''L. viridescens'', and ''L. fermentum''. Some strains of sensitive species may still be insensitive to the toxin from certain strains of ''L. acidophilus''. The toxin does not affect a wide range of bacteria, nor yeast species <ref>[https://www.ncbi.nlm.nih.gov/pmc/articles/PMC242543/ Detection and activity of lactacin B, a bacteriocin produced by Lactobacillus acidophilus. S F Barefoot and T R Klaenhammer. 1983.]</ref><ref>[https://link.springer.com/article/10.1007/s12602-017-9326-2 Lack of Heterogeneity in Bacteriocin Production Across a Selection of Commercial Probiotic Products. J. W. Hegarty, C. M. Guinane, R. P. RossC. Hill, P. D. Cotter. 2017.]</ref>. | + | While most species of ''Lactobacillus'' do not produce bacteriocins, many strains of ''L. acidophilus'' are well known for being able to produce bacteriocins, including probiotics and yogurt strains. Bacteriocins are similar to the [[Saccharomyces#Killer_Wine_Yeast|toxins that some wine yeast strains produce]], however bacteriocins tend to target other species that are closely related to the toxin-producing species (although there are some exceptions to this in the literature). The bacteriocin that ''L. acidophilus'' produces is a narrow spectrum class II bacteriocin, lactacin B (narrow spectrum means that this toxin kills a very narrow range of closely related Gram-positive bacteria). Species that are susceptible to the lactacin B toxin include species that are genetically closely related to ''L. acidophilus'': ''L. leichmanii'', ''L. bulgaricus'', ''L. delbruekii'', ''L. lactis'', and ''L. helveticus''. Species that are insensitive to the toxin are ''L. plantarum'', ''L. casei'', ''L. viridescens'', and ''L. fermentum''. Some strains of sensitive species may still be insensitive to the toxin from certain strains of ''L. acidophilus''. The toxin does not affect a wide range of bacteria, nor yeast species <ref>[https://www.ncbi.nlm.nih.gov/pmc/articles/PMC242543/ Detection and activity of lactacin B, a bacteriocin produced by Lactobacillus acidophilus. S F Barefoot and T R Klaenhammer. 1983.]</ref><ref>[https://link.springer.com/article/10.1007/s12602-017-9326-2 Lack of Heterogeneity in Bacteriocin Production Across a Selection of Commercial Probiotic Products. J. W. Hegarty, C. M. Guinane, R. P. RossC. Hill, P. D. Cotter. 2017.]</ref>. |
==See Also== | ==See Also== |
Revision as of 22:34, 27 September 2017
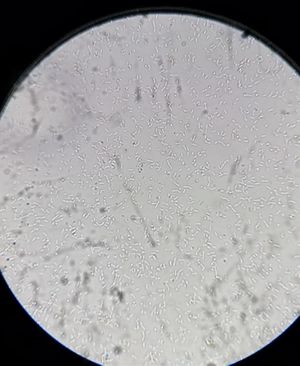
Lactobacillus (often referred to by brewers as "Lacto") is a genus of Gram-positive, rod-shaped lactic acid bacteria (LAB) which produces acidity and sour flavors in the form of lactic acid and secondary metabolites found in lambics, Berliner Weiss, sour brown ales, and gueuze. All Lactobacillus species are facultative anaerobes, which means they grow anaerobically but can also grow in the presence of oxygen and use oxygen to some degree [1]. There are more than 100 species, many of which are found in the human gastrointestinal track [1][2]. In addition to beer, some species of Lactobacillus are also used to ferment yogurt, cheese, sauerkraut, pickles, wine, cider, kimchi, cocoa, and kefir [3]. Lactobacillus can form a pellicle (need reference). See Pediococcus, Brettanomyces, Saccharomyces, and Mixed Cultures charts for other commercially available cultures. See the Sour Worting and Mixed Fermentation pages for brewing techniques with Lactobacillus. See the Alternative Bacteria Sources section for culturing Lactobacillus from grains, yogurt, probiotics, and other sources.
Contents
Commercial Lactobacillus Cultures
Culture Charts
Name | Mfg# | Taxonomy | CO2 Producer (Het/Hom) | Starter Note | Fermentation/Other Notes |
---|---|---|---|---|---|
Bootleg Biology | Sour Weapon L | Lactobacillus plantarum (blended strains) | Facultatively heterofermentative | If you’re looking to drop the pH of wort as quickly and low as possible, Sour Weapon L is your go to Lacto blend. At 98F, we’ve had trial batches drop the pH of wort to 3.0 after just 24 hours. When pitched at 84F, pH should reach 3.5 in 24 hours. This is the ideal bacteria blend to use for acidifying wort for quick/kettle sours, and is also very effective when co-pitched with a yeast strain. As with any Lactobacillus culture, we do not recommend using in worts with 10 or more IBUs as that will prevent significant souring. Isolated from from traditional Norwegian Kveik [4]. | |
Brewing Science Institute | L. delbrueckii | Lactobacillus delbrueckii | Homofermentative | A Lactobacillus bacteria that produces a clean lactic sourness. | |
Craft Cultures | CCYL510 | L. delbrueckii | Homofermentative | Lactic acid bacteria producing moderate acidity and sour flavors found in Lambics, Berliner Weiss, and Sour Ales. Commercial pitches only. | |
Craft Cultures | CCYL512 | L. brevis | Heterofermentative | Typically produces more lactic acid than Lactobacillus delbrueckii. Commercial pitches only. | |
Escarpment Laboratories | Lactobacillus Blend | L. brevis and L. plantarum | Heterofermentative | This blend is designed to be usable at a wide range of temperatures, and is especially suited for kettle souring/sour worting. We recommend pre-acidifying wort to 4.5 with lactic acid, then pitching the Lactobacillus blend in a CO2-purged kettle or fermentor at 32-42°C. | |
Escarpment Laboratories | Lactobacillus brevis | L. brevis | Heterofermentative | This strain is moderately hop-tolerant, and as such it can also be used for long-term souring of <10IBU beers. It also performs well in kettle souring/sour worting where fast and clean lactic acidity is desired. We recommend pre-acidifying wort to 4.5 with lactic acid, then pitching the Lactobacillus blend in a CO2-purged kettle or fermentor at 35-45°C. | |
Inland Island Yeast Laboratories | INISBC-991 | L. brevis | Heterofermentative | Produces more lactic acid at higher temperatures and in low hop worts. 70-95 F Temperature Range | |
Inland Island Yeast Laboratories | INISBC-992 | L. delbruekii | Homofermentative | Produces more lactic acid at higher temperatures and in low hop worts. 70-95 F Temperature Range | |
Inland Island Yeast Laboratories | INISBC-932 | L. fermentum | Heterofermentative | ||
GigaYeast | GB110 | L. delbrueckii? [5] | Heterofermentative | For a 5 gallon batch of beer use 2 liters at 1.040 with high quality yeast nutrient. Keep as close to 86°F (30°C) as possible for 3-4 days with frequent rousing (no stir plate) [6]. | Lactic Acid Bacteria are inhibited by hops, high gravity and low temperatures. You can adjust sourness by increasing or decreasing these variables. More than 7 IBU, gravity above 1050 or temps below 65 F will increase the time to sour or lead to reduced overall souring. Contains ~200 billion cells per homebrew pitch [7].
We recommend brewing with GB110 in one of three ways. I) “Hot Start”: Pitch GB110 to wort at 98 F with little or no hops for 48-72 hrs. Wort may be soured before kettle boil or after. If soured before kettle boil, boil with hop additions as usual. If soured after kettle boil cool wort and pitch yeast. II) “Co-Pitch”: Pitch GB110 into a primary with yeast of your choice at 68-72 F. Wort that is less than 1050 and 7 IBU will typically be very sour in 2-3 weeks. III) “Secondary”: Pitch GB110 after primary fermentation for an aged sour. Souring by this method typically requires several months. Adding simple sugars or fruit etc. will enhance souring in the secondary [8]. Sometimes referred to as GigaYeast's "Fast Acting Lacto". This strain is hop sensitive [9]. |
Lallemand | WildBrew Sour Pitch | L. plantarum [10] | Facultatively homofermentative | See this information from Scott Lucas on MTF. This culture comes in a dry (desiccated) format. Although the manufacturer's website claims this strain is tolerant of 8 IBU, we recommend that brewers treat this strain like any other strain of L. plantarum and do not expose it to any hops until the desired acidity has been produced (for example, see Wort Souring). Recommended temperature: 86-104°F. | |
Omega Yeast Labs | OYL-605 | L. brevis, delbrueckii, and plantarum blend | Hetero/Hetero [11] | 1 liter starter for a 5 gallon batch of beer at room temperature for 24-48 hours. No stir plate unless kept anaerobic. | Quick souring. Pitch into 65°F-95°F [12]. Holding temperature is not required. No longer contains delbruekii [13]. Don't use any hops if possible. 2 IBU is a good target if hops must be used [14]. Contains ~150 billion cells per homebrew pitch [7]. |
RVA Yeast Labs | RVA 600 | L. rhamnosus GG | Homofermentative | No starter necessary per RVA | Homofermentative Lacto strain found in probiotics; sensitive to hops; does well at room temperature. |
SouthYeast Labs | Lactobacillus 1 | Unknown | Heterofermentative | Source: Spontaneously infected beer (South Carolina). Best suits Light sours, gose, farmhouse saison (medium/high acidity). | |
SouthYeast Labs | Lactobacillus 2 | Unknown | Homofermentatative | Source: Prickly pear fruit (South Carolina). Best suits strong sours, and lambic (high acidity). | |
The Yeast Bay | Lactobacillus Blend | L. plantarum, L. brevis, and an unidentified Lactobacillus species | Heterofermentative | The Lactobacillus Blend includes three strains: Lactobacillus plantarum, Lactobacillus brevis and a strain of Lactobacillus isolated from a very unique brewer of American sour beers the returned a sequencing result of "uncultured Lactobacillus". Sure to please anyone with a knack for creating sour beers, it can quickly produce acidity across a wide range of temperatures. This blend can be used on its own for kettle souring prior to pitching yeast to create acidity quickly, or co-pitched with yeast to create sourness over time. It will produce a pronounced and rounded acidity that is the foundation of any complex sour beer. We recommend holding the IBU on the low end (< 2-3) if you'd like to use this blend to create acidity in a shorter time frame. Higher IBUs may result in very slow or no souring (testing is still ongoing to determine IBU at which lactic acid production is inhibited). Temperature: 70-90°F. Cell count: 50-80 million cells/mL (1.75-2.8 billion cells for 35 mL homebrew vials) [15][16]. Recommended temperature range for fastest acid production for kettle souring is 85-90°F, although if kept in the 70's it should produce good acidification in 48-72 hours. A major drop off of in acid production is seen above 90°F [17]. | |
The Yeast Bay | TYB282 | L. brevis | Heterofermentative | TYB282 is a single strain of Lactobacillus brevis isolated out of an unintentionally soured golden ale produced by a Mexican craft brewery.
This strain produces a nice, clean lactic acidity (down to ~pH 3.16-3.18) in unhopped wort within 36 hours at a temperature of ~72-77 F. The higher the temperature (up to 90 F is what we've tested), the faster the acid production. This is a great strain for kettle souring, as it grows rather quickly and produces acidity fast with no detectable off flavors. We are about to begin some trials in hopped wort to test out the acidification in the presence of hop compounds, though we presume there is some level of adaptation to hop compounds given the environment in the beer from which we cultured the strain. Temperature: 70-90 ºF. | |
White Labs | WLP677 | L. delbrueckii (potentially misidentified) | Heterofermentative [11][18] | no stir plate, room temp | Incubate at > 90°F and < 117°F for 5-7 days for greater lactic acid production. Cell count: 50-80 million cells/mL (1.75-2.8 billion cells in a 35 mL homebrew vial) [15]. Not a good strain for kettle souring, but can produce a "soft" acidity over a longer period of time [19]. White Labs claims that it is tolerant to up to 20 IBU, although growth starts to become inhibited at 15 IBU [20][21]. Generally heat tolerant, but sours faster between 100-110°F [20] |
White Labs | WLP672 | L. brevis | Heterofermentative [11][22] | No stir plate, room temp | Produced by The Yeast Bay. More hop tolerant than other Lacto strains, however TYB advises to use wort with less than 10 IBU. White Labs data sheet shows that growth is inhibited to 82% at 5 IBU, and 60% at 10 IBU [20]. Temperature range: 70-95°F (greatly inhibited at 110°F) [20]; 80% attenuation (this may not reflect actual attenuation of wort in a real brewery; see reference [23]). [24] Cell count: 50-80 million cells/mL (1.75-2.8 billion cells for 35 mL homebrew vials) [15]. |
Wyeast | 5335 | L. buchneri | Heterofermentative [11] | 1 liter starter for a 5 gallon batch of beer, 1.020 DME sterile wort, no stir plate, no O2, starter at 90°F if possible 5-7 days | Incubate at 90°F for 5-7 days for greater lactic acid production. Cell count: 1.0 x 108 (100 million) cells/mL (10 billion cells in a 100 mL homebrew pouch) [25]. |
Wyeast | 5223-PC | L. brevis | Heterofermentative [11][22] | no stir plate, room temp is fine | Heterofermentative (produces lactic acid, ethanol and CO2), more hop tolerant. Does well at room temperature. AVAILABLE ONLY FROM JULY THROUGH SEPTEMBER 2014 (Michael Dawson from Wyeast indicated that this culture may return at some point). Jamie Daly indicated on MTF that he got almost no sourness after 24 hours at 100°F (37.8°C). He lowered the temperature to 90°F-95°F (32.2°C-35°C) for 36 hours, and the pH of the wort went down to 3.29. Thus, Jamie recommends 90°F-95°F (32.2°C-35°C) for 60 hours for better souring; avoid warmer temperatures. He also aerated his starter of L. brevis (2L starter of 1.020 DME) and set it on a stir plate at 95°F [26]. The beer wort was not aerated, and the fermenter was flushed with CO2. These methods need verification. Cell count: 1.0 x 108 (100 million) cells/mL (10 billion cells in a 100 mL homebrew pouch) [25]. |
Manufacturer Tips
Omega Yeast Labs on OYL-605
The following is a statement by Lance Shaner, owner of Omega Yeast Labs:
Lance Shaner: "Full disclosure: I own Omega Yeast Labs. Pitching at 120F is a bad idea with this blend. The bug doing most of the work in this blend is Lactobacillus plantarum. The best temp for plantarum is 80-95F [12]. It does not work [as well] over 105°F [12]. Also, we regularly make a 1 liter starter with the Lacto blend for faster souring. Simply pitch the contents of the pouch into 1 liter of sterile 1.040 wort and let sit for 24 hours at 70-80°F before pitching (no need to stir). Adi Hastings mentioned the imperial stout we just kettle soured. We previously brewed a Berliner using the same method. At 17 hours, pH was at 3.42 and temp was 75°F (original pitch temp was 85°F). At 40 hours, pH was 3.24, at which time we boiled. Lower pH in the Berliner compared to the stout at 17 hours likely has to do with different buffering capacities in different worts."
This blend is very susceptible to hops. It is recommended to not use any hops when souring with this Lactobacillus. If hops must be used (some commercial breweries have to use hops for legal reasons), a maximum of 2 IBU is recommended.
Wyeast on 5335
The following is an excerpt with Jess Caudill, Brewer/Microbiologist, at Wyeast Laboratories, Inc. concerning usage of Wyeast 5335 and making a Berliner Weissbier.
- Use 5335.
- If using our 5335, don’t use ANY hops. You can always blend in some IPA or hopped wort after souring takes place if you really need some bitterness or hop flavor/aroma in the beer.
- From one 5335 pack, make a 1L starter with 1.020 DME sterile wort. No O2! Incubate at 90°F if possible for 5-7 days.
- Brew your 5 gallons of wort. Again… no hops. Sterilize the wort. (No need for sour mashes). Cool to 90°F and add 1L 5335 starter. No O2. Try to maintain 90°F for 5-7 days depending on how sour you want the beer.
- After 5-7 days, cool wort to around 68. Pitch with a low pH tolerant strain such as 1007 or 2124. No O2. Ferment for around 1-2 weeks… until you hit terminal.
- Package beer. If bottle conditioning, use 4021 as a bottling strains. Very tolerant to low pH.
RVA Yeast Labs on RVA 600
From their website: a great lactic acid bacterial strain that will add a pleasant tangy sourness. RVA 600 is a pure culture of Lactobacillus rhamnosus GG which is found in many commercial probiotic products which have been shown in clinical studies to have many beneficial effects. These are homofermentative (only produces lactic acid, no carbon dioxide or ethanol) and are hop-sensitive. For more pronounces souring add before you add your yeast. You can sour to taste then add a yeast strain to outcompete the bacteria. Again, hop sensitive so easy on them…or dry hop the heck out of it! You may also want to experiment with blending sour low hop beer with an ale strain beer [27].
Malachy McKenna: "The amount of bacteria in our homebrew units should eliminate the use of a starter. Pitching on the warm side will speed up the souring but RVA 600, a pure culture of Lactobacillus rhamnosus GG, the first commercially available probiotic propagated for use in brewing, does just fine at room temp. We had originally developed RVA 600 as a mix but fell in love with the pure strain [28].
SouthYeast Labs on Lactobacillus 1 and 2
The L2 strain grows best at 86°F-100°F (30°C-37.7°C), and does not work well over 100°F. Keep IBU's low to none. L1 will likely be discontinued due to high amounts of acetic acid production [29].
White Labs on WLP672
"It is intended for secondary, so you only need to do a starter if you are doing a primary fermentation with it. DME would be the best substrate... Since its a Lacto species, you don't really want to aerate it. A slow stir-plate would be good, to keep it moving, but not much more than that." - Sarah Neel, White Labs, Sales and Customer Service (quoted with permission).
Since WLP672 is meant for long term aging, it is not preferred to use for kettle souring/wort souring. See also 100% Lactobacillus fermentation.
General Advice
Starters and Pitching Rate
In addition to the starter information given in the Manufacturer Tips above, this section includes general advice for Lactobacillus starters for homebrewers and brewers. For growing Lactobacillus in a lab environment, or from an initial grouping of cells from a plate/slant or smaller cell count, MRS media is the most efficient growth media. However, for full pitches of Lactobacillus in beer/wort, brewers probably don't want to add that much MRS media to their beer since MRS media has a distinct odor and smell that would not be desirable in beer [30]. Therefore, growing Lactobacillus in a wort-based starter media is recommended for building full pitches of Lactobacillus. We recommend using an Erlenmeyer flask dedicated to growing Lactobacillus in order to lower the potential for yeast contamination. We also recommend using Samuel Aeschlimann's Starter Procedures. See External Resources for additional starter guides.
A good pitching rate for Lactobacillus is 100-125 billion cells per 5 gallons of wort. Having a manufacturer supply a cell count or doing a cell count manually after growth in a starter, and then pitching an exact cell count is the best approach to achieving the desired pitching rate. However, counting cells of Lactobacillus under a microscope can be difficult to achieve due to the small size of bacteria cells, so starter volumes are generally used instead when talking about pitching rates for Lactobacillus [31]. Pitching ~0.5-1 liter per ~20 liters of wort (~0.75-1 gallon per barrel) of Lactobacillus starter is the general guideline. Note that the exact advisable pitching rates of commercial cultures may differ from manufacture to manufacturer [32], and their recommendations might differ from ours.
The amount of growth that occurs in a starter is an unknown unless the brewer can do a cell count. Matt Miller of Sour Beer Blog and Richard Preiss of Escarpment Yeast Labs advise that if ideal growth (1-2 billion cells/mL) can be achieved (for example by using Samuel Aeschlimann's starter procedure or MRS media), then pitching as little as 100-125 mL of fresh Lactobacillus starter for 5 gallons of beer can achieve desirable acidity within 24 hours [33][7]. Moderately ideal growth in a starter results in around 500 million cells/mL, resulting in a 400 mL starter for 5 gallons, and low growth results in around 100 million cells/mL, resulting in a 2 liter starter for 5 gallons, according to Matt Miller (see Matt Miller's article for more details) [7]. If the amount of growth cannot be accurately determined by cell counting under a microscope, then pitching 0.5-1 liter of fresh Lactobacillus starter culture per 5 gallons of wort ensures that an adequate number of cells will be pitched.
Another thing to consider is that achieving a pH of 4 as fast as possible is advisable for preventing off-flavors from contaminating microbes [34]. Larger pitch rates tend to achieve a lower pH faster [35]. Therefore, unless using Samuel Aeschlimann's starter procedure, pitching 0.5-1 liters of starter for 5 gallons of wort is advisable in general. Even when using Samuel Aeschlimann's starter procedure, over-pitching Lactobacillus is not a concern (pitching an overly massive starter wort could produce undesirable flavors), so the same pitch rate should still be considered unless the brewer is confident that high growth rate has been achieved in the starter. Other factors that might affect the effectiveness of a volume based starter is the species/strain of the Lactobacillus being used, how much yeast contamination has occurred, and how old the Lactobacillus starter is. Some species/strains may require a larger volume of starter, as well as if yeast has contaminated the starter or wort (see 100% Lactobacillus Fermentation). If a Lactobacillus culture is older than 1 month, then a fresh starter should be made. Keeping a separate Erlenmeyer flask for Lactobacillus starters can help to prevent yeast contamination [36], as well as using sterilization equipment such as an autoclave or pressure cooker.
Starter mediums that brewers have used include unhopped DME wort starters and apple juice starters. These tend to be adequate for many brewers. However, Samuel Aeschlimann from Eureka Brewing Blog showed that using DME with a little bit of apple juice, chalk, and yeast nutrients provides close to optimal cell densities that match MRS media cell densities. See Samuel Aeschlimann's Starter Procedures. Specific nutrients that will increase growth is thiamine (vitamin B1), or a combination of thiamine and riboflavin (vitamin B2). For example, it has been shown that thiamine is required in order for L. brevis to efficiently convert pyruvate into lactic acid and ethanol. The addition of these nutrients can help encourage growth [37].
Although more experiments and probably needed, agitation is believed to be an important factor for both yeast and bacteria in general. Gentle stirring on a stir plate or orbital shaker, or frequent gentle manual agitation leads to faster growth and a higher number of organisms. Agitation keeps the microbes in solution. It also maximizes the microbes' access to nutrients and disperses waste evenly. In a non-agitated starter, the microbes are limited to the diffusion rate of nutrients, leading to a slower and more stressful growth [38]. If agitation is not possible for whatever reason, a successful starter can be made without agitation. Sam Aeschlimann reported good success with Lactobacillus starters that are not agitated [39].
Although Lactobacillus are tolerant of oxygen and oxygen usually does not negatively affect their growth (except in the case of L. plantarum, which has been shown to produce small amounts of acetic acid when exposed to oxygen and glucose is not present [40][41]), it is also generally not needed (an exception to this may be L. brevis, which has been shown to increase growth rates in the presence of oxygen [26]). Therefore, it is generally best practice to prevent aerating the starter with an airlock for Lactobacillus starters. If exposure to air occurs, and the starter does not smell like it has been contaminated by the exposure, then the starter can still be used.
- For information on mixed culture starters, see Mixed Culture Starters.
Samuel Aeschlimann's Starter Procedures

Although 100% apple juice or 100% DME starters will "work" for Lactobacillus starters, they do not provide optimal growth conditions. Samuel Aeschlimann from Eureka Brewing Blog ran a set of experiments that found a DME based recipe for starter wort that produces a very high cell density similar to that of MRS media, which provides optimal growth rates for Lactobacillus.
The recipe for this starter wort is: 1.040 SG (10°P) Dried Malt Extract wort with 10% apple juice + 20 grams of chalk (CaCO3) per liter + yeast nutrients. Regarding the use of chalk, it is the preferred buffer because it does not react with CO2 (unlike baking soda), so it won't be consumed by exposure to air due to CO2 production by the Lacto. It also has a pKa (maximum buffering capacity) of around 4.6, which is ideal for Lactobacillus growth. The fact that it easily precipitates out also makes it ideal to use as a buffer [42]. Jeff Mello from Bootleg Biology, Nick Impellitteri from The Yeast Bay, and Bryan from Sui Generis blog suggest that using the smaller amount of 1.5-2 grams of CaCO3 per liter is preferable because that amount is easier to precipitate out of the starter and avoid pitching into the beer (the growth differences from using less chalk has not been tested though) [43][44][45]. To create a 1 liter starter for 20 liters of wort, follow these directions:
- Add 100 grams of DME to around 900 mL of water and heat pasteurize/boil as you would normally do for a starter. This should make 1.040 SG (10°P) starter wort.
- Cool the DME wort to the desired incubation temperature (see step 4), and add 100 mL of pasteurized apple juice, 20 grams of chalk (CaCO3), and half a teaspoon of yeast nutrients. The chalk won't dissolve into solution, so don't worry about it. [46]. Boiling the apple juice might destroy some of the nutrients in the apple juice that assist Lactobacillus in its growth, and since it is pasteurized boiling it is not necessary [47].
- Best practice is that starters should not be aerated, although there may be an exception to this for L. brevis [26]. Some people prefer to stir their starter with an airlock in order to keep the bacteria in suspension, others do not use a stir plate and keep the starter still. One advantage to not using a stir plate at least until the brewer is more familiar with their culture is that the top of the starter will begin to clear when the starter is done if the starter was kept still.
- The starter should be held at the temperature best suited for the culture as shown in the Culture Charts.
- Reference the above Culture Charts for how long the starter should be incubated for before pitching. If a stir plate is not used, one indication that the starter is done will be when the top of the starter begins to clear (turbidity is an indication that the culture is growing, and once the top portion of the starter starts to clear then that is a sign that growth has stopped) [39].
- The chalk is not desirable to pitch into the beer because of its buffering effect. The chalk will sediment within hours of being added to the starter, or if a stir plate is used, a couple of hours after the stir plate is turned off [48][39]. The Lactobacillus should stay in suspension for at least a day or two after the starter is done, so swirling the starter isn't necessary, although it is certainly an option. If the starter is swirled, allow a couple of hours for the chalk to sediment out again. After the chalk sediments to the bottom of the flask, pour all of the liquid from the top of the starter into the wort/beer, and leave the chalk sediment behind. Avoid cold crashing the starter because it can have an adverse effect on the bacteria's health [35][46].
See Lacto Starters, by Bryan of Sui Generis blog for additional information on Lactobacillus starters.
Cell Growth
"I typically grow it by itself anaerobically in MRS media. Seems to work very well and results in good growth. I've personally had the best success with MRS media and in an anaerobic environment, though I know some Lactobacillus strains grow aerobically just fine. The problem with growing lactic acid bacteria is the acid they produce will eventually inhibit their own growth. MRS contains a buffer to help combat the drop in pH as a result of LAB metabolism, which keeps the pH around 6-6.5 (I think) for optimal growth. I usually grow them at 35 C, but sometimes incubator space is at a premium (like right now) and I just [use a stir plate with an airlock]" [49]. - Nick Impellitteri from The Yeast Bay on general Lactobacillus cell growth
Maximum cell densities of Pediococcus and Lactobacillus are around 1-9 billion cells/mL, depending on the available nutrients (amino acids and FAN) in the growth media [50][51]. Cell growth rates concur with a drop in pH and a rise in titratable acidity. Brewer's wort has shown to be a nutritionally adequate growth medium for Lactobacillus. Both growth and the the lowering of pH begin to stabilize around 12-48 hours (assuming the Lactobacillus does not have any yeast to compete with). Titratable acidity will also rise drastically during growth, but will also continue to rise after growth has completed. The maximum growth that a particular species or strain is capable of might be explained by its pH tolerance, and thus its ability to produce more acid. For example, L. plantarum has been shown to grow in a very low pH environment (3.37-3.0 pH, depending on strain) due to their ability to better control large pH gradients between the cytoplasma and the external environment. This has been shown in the above data provided by Matt Humbard, as well as this reference [50]. Thomas Hübbe's masters thesis showed that a strain of L. brevis had a spike of growth after 50 hours, and then a small dip in cell count after 96 hours, at which time the cell count remained consistent for at least 528 hours [52].
Cell growth can also be influenced by the presence of other microorganisms, such as Saccharomyces and Brettanomyces. One study by Hübbe showed that L. brevis and L. parabrevis grew to the normal high cell counts when grown individually and without competition. When co-fermented with Brettanomyces, the cell count of L. brevis was halved, and the growth rate of L. parabrevis was greatly diminished to about 15-20% (the pitching rate of Brettanomyces was also tested, and seemed to not have an effect on the Lactobacillus growth). When co-fermented with both S. cerevisiae and Brettanomyces, the Lactobacillus growth was greatly diminished to about 2-13% of what the normal cell growth was without competition. This appears to correspond with anecdotal reports from brewers that some Lactobacillus species/strains do not compete well with yeast, especially S. cerevisiae [52].
Effects of Oxygen
All prokaryotes, which includes all bacteria, are categorized based on the levels of oxygen in their environment in which they can grow and how they utilize oxygen if at all [2]. Lactobacillus species are usually considered to be "facultative anaerobes" (or "facultative aerobes") [1], however they are a special case. Facultative anaerobes usually make energy from oxygen if it is present via the oxidative phosphorylation pathway, but otherwise engage in anaerobic fermentation [53][54]. Lactobacillus species can utilize oxygen, but not through the oxidative phosphorylation pathway. They use an alternative pathway instead. This pathway uses flavine-containing oxidases and peroxidases to carry out the oxidation of NADH2 using O2 [55][56]. Lactobacilli, therefore, are unique in that they blur the line between facultative anaerobes and another class of prokaryotes known as "aerotolerant anaerobes". Aerotolerant anaerobes do not use oxygen to generate energy but can grow in the presence of oxygen.
The important take away here is that oxygen doesn't significantly affect Lactobacillus species. They do not care if oxygen is present in order to grow and produce energy for themselves and lactic acid for brewers. They also do not produce significant amounts of butyric acid or isovaleric acid in the presence of oxygen [57].
There are, however, a few exceptions to this in the scientific literature. For example, L. plantarum, which is a facultatively heterofermentative species, is homolactic without the presence of oxygen and produces only lactic acid. After food sources have been exhausted and in the presence of oxygen, however, L. plantarum switches to heterolactic fermentation, and further converts lactic acid into acetic acid. In a lab setting the conversion of lactic acid to acetic acid only happened when glucose was no longer available (this may not be the case in wort where other limiting factors such as low pH can prevent L. plantarum from continuing their metabolic processes before all glucose is consumed, which is the case in kettle souring), and only during the stationary phase (after growth stopped). During the conversion of lactic acid to acetic acid, L. plantarum also produces hydrogen peroxide (H202), which is toxic to microorganisms and is thought to be a protection mechanism for L. plantarum [58][40][41][59]. This same process has also been observed in one strain of L. brevis and under similar conditions of depleted nutrients and oxygen [60]. Omega Yeast Labs reports that no noticeable acetic acid is produced if the oxygen is not purged with their OYL-605 Lactobacillus blend which contains L. plantarum, and brewers should not aerate wort during sour but purging O2 is not required [61]. L. brevis has been shown to increase growth rates in the presence of oxygen [26]. Thomas Hübbe's master thesis showed that under lab growth media "VLB-S7-S", "NBB®-A", and MYPG + cyclohexmide, both strains of Lactobacillus tested (L. brevis and L. parabrevis) did not show growth in an aerobic chamber, but did grow in an anaerobic chamber [52].
Hop Tolerance
The following are comments by Bryan of Sui Generis Brewing blog:
There is a fair bit of research into hop tolerance out there; it's not a simple topic as a number of factors come into play to produce hop tolerance. To make things even more complicated, hop tolerance is an inducible trait in many Lactobacillus species - meaning that a seemingly susceptible strain can become resistant by culturing in ever-increasing doses, and a seemingly resistant strain can become susceptible after a generation or four in a hop-free media.
I've been trying to generate a permanently high-alpha acid resistant lacto strain for a few months now. I've been culturing L. brevis in escalating IBU wort (starting at 10, currently at 25). Every 4th generation (1 generation = a subculture of a stationary-phase lacto culture, not as in # cell divisions) I pass it through 2 generations of an IBU-free media to try and select for strains which maintain this resistance. This seems to have worked up to ~18 IBU (update: 20-30 IBU), but past that point the resistance appears to remain inducible. I'm hoping a few more generations will provide me with a permanently tolerant strain. Update: I had made a strong (~80ibu) wort that I diluted with unhopped wort. I would grow the Lactobacillus for a few passages (1 passage = grow culture to completion, then dilute ~1:100 into fresh wort to start next passage) at a set the IBU level, then passage to a wort 3 or so IBU higher. I did 50-60 generations before I got to the high IBU levels (~3 months, 1-2 days per generation). I never did anything to determine how much of that resistance was inheritable [62].
There are some other options; I've purified (but didn't keep - doh) some pretty resistant strains from grain by by making plates where you half-fill a plate, on an angle, with a high-IBU wort, and then overlay that with a no-IBU wort. This gives you a gradient plate, with low-IBUs on the end where the hopped-wort layer is thinnest and high IBUs where it is thickest. Some of those strains were resistant to over 30IBU, but being early in my yeast farming days I didn't bother keeping those [63].
Hops contain multiple compounds which are bacteriostatic. Alpha acids are the best understood, but other compounds such as beta acids, a number of polyphenols (e.g. xanthohumol), and even some of the aromatic oils (e.g. humulene) have been found to have some inhibitory effects on lactobacilli. The later compounds (especially the beta acids) are why aged hops retain inhibitory characteristics, despite being nearly devoid of alpha acids. In all cases these compounds appear to inhibit the bacteria in the same way - all of these compounds contain fairly large, flat-ish, hydrophobic regions. These regions do not "like" to be in water, and thus will be driven into the hydrophobic core of the bacterial plasma membrane. This opens minute holes in the membrane which prevents the bacteria from maintaining ion (in particular, proton) gradients, leading to suppression of growth and even death of the bacteria.
Hop resistance is generally due to the induced expression of "multi-drug transport" (MDT) genes, which are "pumps" that recognize the general chemical signature of membrane-disruptive compounds, and then pump them out of the cell. Other mechanisms may also be involved - a few papers have identified changes in the lipid make-up of the plasma membrane, which may increase stability. This change also occurs in response to alcohol (to improve stability), so its not clear if that particular change has anything to do with hop resistance.
Lactobacilli usually don't have these MDT genes 'on', which is why a lot of strains won't do well with hops in the first batch of beer, but over time become more and more tolerant as they increase expression of the MDT's. The overall MDT expression level, in theory, determines the maximum resistance of the bacteria. In the case of my experiments, I'm looking for mutants whose MDT's are permanently stuck 'on' for the resistant strain and 'off' for the sensitive strain [64].
Hop tolerance is not only species dependent, but is also strain dependent. For example, a dissertation by F.J. Methner measured the pH drop of wort that started at a pH of 5.55 from day 3 to day 14 for several strains of L. brevis at different IBU levels (7,9,11,13 and 18 IBU's). One strain of L. brevis eventually got down to a pH of 3.8 at day 14 with 7 IBU's, while another strain got down to 3.3 pH at day 14 (with other strains in-between those numbers). At 18 IBU, the relatively hop intolerant L. brevis strain got down to only 4.2 pH, while another strain got down to 3.7. In general, the higher the IBU, the slower the pH drop. Interestingly, another species called L. coryniformis was shown to be more hop tolerant than L. brevis. L. coryniformis dropped the 18 IBU wort down to 3.6 pH over 14 days [65].
Methner's data is shown below; graphs created by Benedikt Rausch [65]. Y axis = pH, X axis = days.
- L70: L. coryniformis
- L14, L18, L22, L29, L88, L92: L. Brevis
See also:
- Hops Antimicrobial Properties and Dry Hopping Inhibits Lactobacillus.
- How hops prevent infection, by Lars Garshol.
- Per Buer's Video Demonstration of how dry hopping inhibits Lactobacillus.
- "CLevar" on Ratebeer.com data point on Lactobacillus being inhibited by hops, but not as much by iso-alpha acid hop extract.
Alcohol and Sugar Tolerance
Lactobacillus is generally tolerant of alcohol and high levels of sugar (although growth is diminished once sugar content exceeds 20% due to osmosis stressing the cell wall). In the presence of high amounts of ethanol, the alcohol tolerant strains pack their cell walls with fatty acids, which slows the fluidity of the cell membrane. The alcohol tolerance of Lactobacillus is dependent on both strain and the growth substrate, with alcohol tolerance generally higher when glucose or starch are available. In one study that looked at 31 strains of Lactobacillus, all strains grew effectively at 4% ABV. All of them still grew at 10% ABV, although some strains exhibited difficulty growing effectively at 10% ABV. Growth was diminished in general at 12% ABV (and the few strains that were alcohol intolerant stopped growing), but most still achieved some growth. Eight of the strains tested were still able to exhibit significant growth at 16% ABV, and in general, most strains were able to exhibit at least small growth at 16% ABV (more so on starch and glucose versus cellobiose, lactose, or xylose). In general, the species that were less tolerant of high amounts of ethanol (10-16%) where: L. amylovorous (1 out of 4 strains was particularly intolerant), L. hilgardii (1 strain still grew at 16% ABV, but less than the others), L. pentosus (1 strain still grew on starch medium, but not on glucose above 10% ABV), and L. casei (most strains grew in most growth media, but generally less than other species). In general, the strains of L. brevis and L. plantarum were more tolerant of high ABV concentrations [66].
Peyer et al. (2017) examined the effects of the sugar content of brewer's wort on a strain of Lactobacillus amylovorous. They found that the higher gravity of the wort increased the growth of the Lactobacillus, and therefore also the lactic acid production. This increase was linear until the extract reached 16%, at which time the growth increase began to slow down. The higher growth and more lactic acid production in higher gravity wort was probably due to increased nutrients as well as a higher buffer capacity. This growth increase plateaued in wort that had 18-20% sugar content, which was likely due to osmotic stress on the cells [67].
Tolerance of Extreme Temperature
Most Lactobacillus species have a thermal death rate of ~145°F (63°C). Freezing without glycerol will kill most cells, but it is possible for a very small number of cold-resistant mutant cells to survive [68] (~1:19:00 in).
Storage
For dried Lactobacillus, such as probiotics or Dry Yeast for Sour Ales BlackManYeast products, Bryan of Sui Generis Blog's lab studies have shown that they can lose viability ~80 times faster at room temperature than when stored at refrigeration temperatures. Therefore, it is recommended to store dried Lactobacillus at refrigeration temperatures. Short term storage of liquid cultures (less than 2 months) should also be stored refrigerated. Consider making a starter before using a culture that is not fresh.
Liquid cultures become stressed by two factors: storage in an acidic environment, and storage without sugar [69]. Sugar storage creates more acid as the Lactobacillus ferments it, so it may not be ideal unless the Lactobacillus is continually fed. Ideally for longer term storage, liquid cultures of Lactobacillus should be stored frozen with 20% glycerol, or refrigerated as slants with water or mineral oil. Also, there is anecdotal evidence that certain species may survive better at room temperature. Bryan hypothesizes that stable temperatures may be more important than storing at an "ideal" temperature [70]. For instructions on how to make slants at home capable of storing any microbe for potentially 2+ years, see Bryan video on Sui Generis Brewing (requires a pressure cooker).
A practical option for brewers without a pressure cooker is to store the liquid culture with a few grams of a buffering chemical such as calcium carbonate (chalk), potassium phosphate, calcium sulfate (gypsum), or calcium hydroxide (pickling lime). The exact amounts should be adjusted to reach a pH of about 4.0-6.0 for the entire solution (begin with 1 or 2 grams per liter, and adjust as needed) [71].
Tom Belgrano offers these additional steps in order to remove the residual sugars from a storage solution, as well as raise the pH [72]:
- Cold crash the starter until the wort is clear.
- Decant the liquid, and then refill it with water (distilled is recommended, but not required).
- Cold crash again until the liquid falls clear.
- Decant the liquid.
- Store the resulting slurry at refrigeration temperatures. A slight drop in pH may still occur, but this technique should keep the storage solution well above 4.0 pH.
Commercial brewers who are attempting to re-use pure Lactobacillus cultures for kettle souring will often pull a portion of the soured wort before boiling it. The soured wort with living Lactobacillus is stored for later use in future batches. Cold storage is probably preferred. This can be difficult to accomplish because the residual sugars in the wort can easily attract a yeast contamination or other contaminations (see 100% Lactobacillus fermentation) [73]. Storing buffered wort above 4.5 pH anaerobically could provide the potential for botulism toxin formation (see this Beer and Wine Journal article by Dr. Chris Colby). Autoclaving, pressure cooking, or tyndallizing the wort before adding the Lactobacillus culture to it would provide a sterile media free of potential botulism spores [74], but may not be a practical process for the brewer. It might be possible to achieve a pH of 4.0-4.5 using CaCO3 (chalk) to buffer the pH in a range that extends the viability of the Lactobacillus, and also makes the wort safe for storage (this needs more data) [75] (amounts of CaCO3 needed). Using chalk as a buffer would be similar to using the Eureka starter method above; the chalk should settle to the bottom of the vessel, and can be decanted off of.
Selecting for on Agar
Lactobacillus species can be isolated on agar plates by using a selective media called Rogosa SL. Rogosa SL agar contains ammonium citrate, sodium acetate, a relatively low pH, and acetic acid, which select for Lactobacillus while inhibiting mold and other bacteria. This medium will not inhibit yeast on it's own, however. If inhibiting yeast is a requirement then add 10 mg/L cycloheximide to inhibit most yeast [76].
- See Rogosa SL Agar (Lactobacillus); Basement Brew Lab, by Dustin Metzger for more information.
Effects on Mixed Fermentation
The presence of Lactobacillus can stall or slow yeast fermentation. This is likely a combination of low pH as well as the ability of lactic acid to change the way yeast ferments. Normally, yeast will ferment glucose first before any other sugars that are also available. The presence of lactic acid appears to change the way yeast ferments by allowing them to consume multiple types of sugars regardless of whether or not glucose is present. See Lactic Acid for more information.
Peyer et al. (2017) observed that growth of US-05 was 82% at a pH of 3.51, and 53% at a pH of 3.17. Fermentation was delayed by 2-4 days (the lower the pH, the longer the start of fermentation was delayed). In a co-fermentation of Lactobacillus amylovorus and US-05, the initial growth of the L. amylovorus continued for 3 days while the US-05 was delayed. On day 7, the US-05 recovered and continued growth, and the growth of the Lactobacillus was slowed starting on day 5. This was due to the increase in ethanol from fermentation, lower pH, and the depletion of nutrients for the Lactobacillus. It is also possible that the yeast benefited from the autolysis of the Lactobacillus, which is speculated to have released nutrients that were made available to the yeast [67].
Also found was an increase in diacetyl and acetoin in the beers that were co-fermented with L. amylovorus and US-05 versus the beers that were kettle soured or mash soured. Both of these compounds are responsible for the buttery taste in beer. Normally, after primary fermentation the yeast reduces daicetyl to acetoin, which is then converted to butanediol, however during a co-fermentation with Lactobacillus, this conversion was inhibited in this study [67].
Sensorily speaking, the kettle soured beer tasted "more pure" with less off-flavors, and a plum-like aroma. The sour mash and kettle sour beers had a lingering sour aftertaste, while the beer co-fermented with L. amylovorus and US-05 was described as having an astringent aftertaste. This astringent aftertaste was speculated by the authors to be caused by LAB cell autlysis, which might have also contributed to a more complex flavor profile in the co-fermented beer [67].
Commercially available Lactobacillus strains and their pH change over time
All data provided by Matt Humbard. Similar results were reported by Lance Shaner's 100% Lactobacillus Fermentation experiment.
pH change at 86°F

Time (hours) | Wyeast Lactobacillus buchneri | White Labs Lactobacillus brevis | White Labs Lactobacillus delbrueckii | Omega Lactobacillus plantarum |
---|---|---|---|---|
0 | 5.9 | 5.9 | 5.9 | 5.9 |
6 | 5.57 | 5.13 | 5.59 | 4.81 |
18 | 4.4 | 3.94 | 4.62 | 3.6 |
24 | 4.2 | 3.78 | 4.6 | 3.45 |
75.5 | 3.88 | 3.44 | 4.46 | 3.27 |
120 | 3.85 | 3.4 | 4.4 | 3.25 |
pH change at 98°F

Time (hours) | Wyeast Lactobacillus buchneri | White Labs Lactobacillus brevis | White Labs Lactobacillus delbrueckii | Omega Lactobacillus plantarum |
---|---|---|---|---|
0 | 5.9 | 5.9 | 5.9 | 5.9 |
6 | 5.61 | 5.05 | 5.55 | 5.02 |
18 | 4.15 | 3.74 | 4.54 | 3.22 |
24 | 4.05 | 3.67 | 4.54 | 3.2 |
75.5 | 3.5 | 3.31 | 4.32 | 3.22 |
120 | 3.5 | 3.29 | 4.3 | 3.21 |
pH change at 102°F

Time (hours) | Wyeast Lactobacillus buchneri | White Labs Lactobacillus brevis | White Labs Lactobacillus delbrueckii | Omega Lactobacillus plantarum |
---|---|---|---|---|
0 | 5.9 | 5.9 | 5.9 | 5.9 |
6 | 5.45 | 5.02 | 5.5 | 5.11 |
18 | 4.13 | 3.73 | 4.61 | 3.4 |
24 | 4.06 | 3.6 | 4.58 | 3.29 |
75.5 | 3.72 | 3.33 | 4.47 | 3.23 |
120 | 3.68 | 3.3 | 4.4 | 3.2 |
pH change at 108°F

Time (hours) | Wyeast Lactobacillus buchneri | White Labs Lactobacillus brevis | White Labs Lactobacillus delbrueckii | Omega Lactobacillus plantarum |
---|---|---|---|---|
0 | 5.9 | 5.9 | 5.9 | 5.9 |
6 | 5.6 | 4.95 | 5.51 | 4.99 |
18 | 4.49 | 3.71 | 4.8 | 3.54 |
24 | 4.37 | 3.52 | 4.6 | 3.45 |
75.5 | 4.33 | 3.35 | 4.62 | 3.34 |
120 | 4.29 | 3.3 | 4.51 | 3.3 |
Metabolism
- Editor's note: the following section was reviewed for accuracy by MTF members Bryan of Sui Generis Blog, Matt Humbard, Lance Shaner, and Richard Preiss.
Types of Metabolism
All metabolism by Lactobacillus, including growth, will require sugar to be consumed and lactate (lactic acid) to be produced. Two categories of metabolism exist, homolactic and heterolactic. In summary, homolactic fermentation produces only lactic acid, while heterolactic fermentation produce lactic acid, CO2, and ethanol/acetic acid [78].
Homolactic
Homolactic metabolism is described as the cell catabolizing one molecule of glucose to yield two molecules of pyruvate, which is then further reduced to two molecules of lactate (lactic acid). Homolactic fermentation only allows the fermentation of hexoses (glucose, mannose, etc.). Homolactic metabolism follows the Embden-Meyerhof-Parnas pathway [77].
Heterolactic
Heterolactic metabolism is described as the cell catabolizing one molecule of glucose into one molecule of CO2, one molecule of glyceraldehyde phosphate, and one molecule of acetyl phosphate. The molecule of glyceraldehyde phosphate is reduced to one molecule of lactate, and the acetyl phosphate is reduced to one molecule of ethanol (or one molecule of acetic acid instead of ethanol, depending on its growing environment [79]). Heterolactic fermentation allows the fermentation of hexoses and pentoses [80]. Heterolactic fermentation follows the Phosphoketolase Pathway, which is a branch of the Pentose Phosphate Pathway (also called the "Phosphogluconate Pathway") [77][81][82][83]. When different substrates are available to heterolactic fermenting Lactobacillus, such as fructose or oxygen, acetate (acetic acid) can be produced instead of ethanol [50].
Categories of Lactobacillus
There are three categories of Lactobacillus based on the type of fermentation they are capable of (homolactic, heterolactic, or both).
- Obligatory homofermentative Lactobacillus only perform homolactic fermentation, and thus only produce lactic acid [77].
- Obligatory heterofermentative Lactobacillus only perform heterolactic fermentation, and thus produce lactic acid, CO2, and ethanol (or sometimes acetic acid instead of ethanol) [77].
- Facultatively heterofermentative Lactobacillus generally are homolactic when there is an abundance of carbohydrates, but can also perform heterolactic fermentation when carbohydrates are not abundant [77].
Other factors can determine if a facultative heterofermentative species uses homolactic or heterolactic fermentation. For example, L. plantarum, which is a facultatively heterofermentative species, is homolactic without the presence of oxygen. In the presence of oxygen, however, it performs heterolactic fermentation, and produces acetic acid [40][41][59].
Obligatory Homofermentative [84] | Obligatory Heterofermentative | Facultatively Heterofermentative |
---|---|---|
L. acidophilus | L. brevis | L. casei |
L. delbruekii | L. buchneri | L. curvatus |
L. helveticas | L.fermentum | L. plantarum |
L. salivarius | L. reuteri | L. sakei |
L. rhamnosus [85] | L. pontis | L. bavaricus [86] |
L. lactis [86] | L. cellobiosus [86] | L. coryniformis [86] |
L. leichmannii [86] | L. confusus [86] | |
Streptococcus bovis [86] | L. coprophilus [86] | |
Streptococcus thermophilus [86] | L. fermentatum [86] | |
Pediococcus acidilactici [86] | L. sanfrancisco [86] | |
Pediococcus damnosus [86] | Leuconostoc dextranicum [86] | |
Pediococcus pentocacus [86] | Leuconostoc mesenteroides [86] | |
Enterococcus faecium [86] | Leuconostoc paramesenteroides [86] | |
Enterococcus faecalis [86] |
Sugar Utilization
Lactobacillus generally prefers glucose, fructose, and maltose, and does not ferment maltotriose. Some species may prefer certain types of sugars over others. For example L. plantarum ferments glucose first, and then fructose if it is available. L. reuteri ferments maltose first, while L. brevis feeds on maltose, glucose, and fructose. Disaccharides such as sucrose and maltose enter the cells through specific types of membrane transport proteins called permeases, and are broken down into monosaccharides through phosphorolysis before they enter the normal carbohydrate metabolic pathway [87]. Peak sugar consumption without competition from yeast is typically 48 hours, and very little alcohol or CO2 is produced (around 0.10-0.30% ABV, far less than the 0.5% required for non-alcoholic drinks). Consumption of sugars occurs mainly during the 48 hour growth period, but also occurs after growth has stopped. No more than 0.5-1°P worth of sugar is consumed by Lactobacillus. Rather than high residual sugar concentration being the limiting factor on growth it is thought that low pH and other metabolic byproducts weaken and finally stop the growth of Lactobacillus [50]. For a chart and in depth discussion on what types of sugars are fermentable by different species of Lactobacillus, as well as charts on secondary metabolites, see Matt Humbard's Physiology of Flavors in Beer – Lactobacillus Species blog article.
A small number of strains of Lactobacillus can also break down polysaccharides and starches. They are referred to as "amylolytic LAB". They generally belong to the species Lb. manihotivorans, L. fermentum, L. amylovorus, L. amylophilus, L. plantarum or L. amylolyticus. This seems to be associated with a gene called "amyA", which encodes for extracellular alpha-amylase activity, as well as alpha-glucosidase, neopullulanase, amylopectin phosphorylase, and maltose phosphorylase. This activity is limited by high amounts of glucose, maltose, or sucrose [87]. Some species can also produce beta-glucosidase capable of breaking down monoglycosides (see Glycosides), but not diglycosides. The activity of both alpha and beta-glucosidase enzymes are stable at low pH ranges of 3-4, are generally encouraged by increasing percentages of alcohol all the way up to 12% v/v, and are optimal at 35-45°C (depending on strain) [88].
100% Lactobacillus Fermentation
Lance Shaner's experiment on testing 100% Lactobacillus Fermentation showed that pure cultures of WLP677, WLP672, Wyeast 5335, Wyeast 5223-PC, and the L. plantarum from Omega Yeast OYL-605, could not fully attenuate a 1.037 SG wort. The most attenuative Lactobacillus culture, WLP677, was only able to attenuate down to 1.03255 SG. It is likely that all species and strains of Lactobacillus available to brewers cannot fully attenuate wort. In addition, this study showed at most a 0.29% ABV in 100% Lactobacillus fermentations (attributed to WLP677). See 100% Lactobacillus Fermentation for more information. If a higher attenuation is achieved, cross contamination of yeast is most likely the cause. Thomas Hübbe's masters thesis also supports that Lactobacillus attenuates less than 10% of the sugars in wort [52].
The amount of CO2 produced is very small in heterofermentative species. Lance Shaner of Omega Yeast Labs noted that although L. brevis is classified as obligatory heterofermentative, the human eye cannot detect any CO2 production in the Omega Yeast Lactobacillus blend (OYL-605). Lance still needs to test this blend to see if it produces any CO2 at all. There have been reliable reports of pure Lactobacillus brevis cultures producing a layer of bubbles on the surface of wort if roused [89]. It is clear though that any type of Lactobacillus, regardless of whether it is heterofermentative or homofermentative, cannot produce a krausen. Krausens are sometimes seen even with the use of commercially available Lactobacillus cultures and good sanitation techniques. If a krausen develops in wort when it is the only culture that is pitched, this is indicative of cross contamination of Saccharomyces or Brettanomyces in either the wort, or the Lactobacillus culture itself [90]. In addition to this, heterolactic fermentation by Lactobacillus can only produce 10-20% of the ethanol that Saccharomyces can produce [91], therefore a high level of attenuation cannot be achieved by Lactobacillus and is again a sign of cross contamination by yeast.
Recent studies on lactic acid fermented malt beverages shows that Lactobacillus produces only about 0.1% ABV, producing "non-alcoholic" fermented malt beverages [92][50]. Elde Arendt, a brewing scientist that specializes in Lactobacillus presented her work at the Belgian Brewing Conference 2015. In it she explained that LAB will only ferment 0.5°P of wort regardless of the gravity of that wort. When asked at the end of the presentation why Lactobacillus only ferments ~0.5°P (note that Shaner's experiment shows Lactobacillus fermenting ~1°P, although this may be due to a margin of error since Shaner only performed this experiment once), considering that Lactobacillus ferments maltose and there is plenty of maltose in wort, Arendt responded that she believes that the bacteria reaches max cell density in the wort with relatively little sugar requirements (~16 mins in and ~25 mins in):
- See also 100% Lactobacillus Fermentation.
Primary/Secondary Metabolites
Primary Metabolites
Lactic acid is the primary metabolite for Lactobacillus, as well as CO2 and ethanol/acetate (acetic acid) in heterofermentative species. Acid production is at it's highest during the exponential growth phase, but continues into the stationary and decline phases. Typically just under 50% of the lactic acid produced is L-lactic acid (more nutritionally relevant) while the slight majority is D-lactic acid [50]. The amount of lactic and acetic acids produced varies from species to species. For example, the referenced study showed that L. plantarum produces more than twice the amount of lactic acid than L. brevis, and L. reuteri produced slightly more lactic acid than L. brevis. L. reuteri produced around twice as much acetic acid than L. brevis, and L. plantarum produced very little acetic acid. The small amount of acetic acid produced by L. plantarum in this study was explained by oxygen exposure during sampling, while the obligate heterofermentative species (L. reuteri and L. brevis) produced acetic acid as a direct result of their heterolactic fermentation [50].
Secondary Metabolites
Both primary and secondary metabolites play a large role in the flavor and aroma profile of wort fermented with Lactobacillus. Secondary metabolites are compounds that are not directly related to the growth of an organism, but often assist with survival [93]. These secondary metabolites are produced by the pathways mentioned above, and different strains probably regulate the enzymes involved in various pathways differently and produce different secondary metabolites [94]. Thus, different species and strains can produce a wide variety of flavors and aromas (compare this to food grade lactic acid in which none of these secondary metabolites exist). These secondary metabolite are the result of carbohydrate fermentation and amino acid metabolism [87]. Major secondary metabolites that
An example from one study showed that L. plantarum produced significantly more diacetyl, acetoin (yogurt-like flavor), and acetaldehyde than L. reuteri and L. brevis. These three compounds were associated with dairy-related notes of "buttery", "lactic", and "yogurt" flavors identified during sensory testing [50]. Some LAB can release these compounds through the catabolism of citric acid, which is found in wort. Ester production is generally insignificant, although significant ester formation has been found during malolactic fermentation in red wines, and ethyl acetate has been found to be produced in malt based beverages [87]. Some strains may also produce fusel alcohols and other off-flavors. For example the referenced study found an accumulation of the fusel alcohol n-Porponal in the sample of L. reuteri, and a small decrease of isovaleric acid coupled with a small increase of hexanoic acid by L. brevis, L. plantarum, and L. reuteri (only 0.25-0.32 mg/L was found, and the flavor threshold of hexanoic acid is 5.4 mg/L [95]) [50]. Heterofermentative species can also produce tetrahydropyridines (THP), which is the cause of "mousy" off-flavors [96]. Aldehydes (2-methyl-1-propanal, 2-methyl-1-butanal, 3-methyl-1-butanal) and their associated non-fusel alcohols (2-methyl-1-propanol, 2-methyl-1-butanol, and 3-methyl-1-butanol) can be produced from amino acids such as leucine, isoleucine, and valine to form fruity flavors [87]. A few species, especially most strains of L. fermentum, and some strains of L. delbrueckii subsp. bulgaricus, can produce ropiness in the form of exopolysaccharides, similar to Pediococcus [87].
Dongmo et al. (2017) found 56 volatile flavor compounds, including various esters, alcohols, ketones, aldehydes, acids, ethers compounds, sulfur compounds, heterocyclic compounds, phenols, terpenes, lactones, and several unidentified compounds. Key compounds produced by Lactobacillus include acetaldehyde (thought to be a major flavor contributor to kettle soured beers [67]), β-Damascenone, furaneol, phenylacetic acid, 2-phenylethanol, 4-vinylguaiacol, sotolon, methional, vanillin, acetic acid, nor-furaneol, guaiacol and ethyl 2-methylbutanoate. Acetaldehyde was the most impactful aroma compound found followed by propan-1-ol and γ-dodecalactone. Acetaldehyde was generally produced in much higher amounts (~23-64 µg/L) by the select strains of L. plantarum, while L. amylolyticus and L. brevis produced only 1.5-3 µg/L. In fact, the levels of all of these compounds differed significantly based on the species and strain. The selected strains of L. brevis were associated as having worse aromas that were dominated by methional (cooked potatoes), acetic acid (vinegar), and nor-furaneol (caramel-like). The L. plantarum strains selected were identified as producing more positive aromas from compounds such as β-damascenone (apple/fruit juice), furaneol (strawberry), 2-phenylethanol (rose/caramel) and ethyl 2-methylbutanoate (citrus) Small but significant amounts of linalool and geraniol were also found, which are normally terpenes found in hops. Vanillan is formed from ferulic acid by some Lactobacillus species as well as Oenococcus oeni [92].
The type of grain that the Lactobacillus is fermented in may also play a role in the types and amounts of secondary metabolites that are produced. One study compared volatile acids produced by a probiotic strain of L. plantarum (NCIMB 8826) when fermented in oats, barley, malted barley, and wheat. In oats, there was slight increase in oleic acid and linoleic acid and a decrease when fermented in wheat, barley, or malted barley. In malted barley, there were small increases in flavor active compounds such as furfural ("almond" flavor), 2-ethoxyethyl acetate and isoamyl alcohol, but little to none detected when fermented in oats, wheat, or unmalted barley. Acetic acid production was higher in barley and malted barley than it was in oats and wheat. Many other organic acids in the oats, wheat, barley, and malted barley were supposedly taken up by the L. plantarum during fermentation. In barley, there were trace amounts of new acids created that were not already in the barley itself [97]. Some species of Lactobacillus, including L. lactis and L. plantarum, produce diacetyl (which can be reduced to acetoin and 2,3-butanediol) as an intermediate metabolite from consuming sugar, citrate, and amino acids. However, citrate levels are rather low in malted barley (but higher in sorghum), and diacetyl production has been observed to be very low in barley and oat based worts [87].
Aging has a large impact on the aromas and flavors produced by Lactobacillus fermentation over time and is typically influenced by temperature of the environment, oxygen exposure, and the byproducts of fermentation. Generally, fermentation has a positive effect on preserving some aroma and flavor compounds. Other compounds may change, causing aroma and flavor changes. For example, one study characterized wort freshly fermented with L. plantarum as "butter" and honey", and when aged as "yogurt" and "sour". In the same study, L. reuteri was characterized as "sour" when fresh, and "honey" and "pungent" when aged. L. brevis was characterized as "soy sauce" when fresh, and "yeasty" and "cider" when aged [50].
Many strains of Lactobacillus and other lactic acid bacteria can produce tannase, which is an enzyme that breaks down a certain class of tannins called "hydrolizable tannins" (for example, tannic acid). The enzymatic breakdown of tannins provides a food source for the Lactobacillus. In the cited study, a strain of L. plantarum was selected out of 47 other tannase producing LAB as being the highest producer of this enzyme. Although the optimum pH for tannase is 5-8, it is also at least 50% active at a pH of 3-7 and a temperature of 15-30°C. Tannase has been produced as a product for removing haze in food products such as iced tea, wine, and beer [98][99]. Some Lactobacillus strains could therefore have a positive effect on beer clarity by breaking down some haze forming tannins [100].
See the Elke Arendt video presentation above on the referenced study, starting at ~14:45.
In summary:
- Different species of Lactobacillus are capable of fermenting different types of sugars, including sugars that Saccharomyces may not be able to ferment.
- All types of Lactobacillus produce different levels of secondary metabolites (compounds that are not required for the organism to live [101]) in addition to the primary metabolites discussed above. These include acetaldehyde, diacetyl, fusel alcohols, and many more compounds (see Matt's article for more details).
- Despite some beliefs by brewers, Lactobacillus has not been shown to produce significant levels butyric acid (see the butyric acid page for more information) in the presence of oxygen or otherwise in brewer's wort. Some species might be able to produce small amounts of Isovaleric Acid in the presence of Streptococcus thermophilus or another alpha-keto acid producing microorganism (see the Isovaleric Acid page for details).
- Although Lactobacillus are not not inhibited by oxygen, they are generally not considered to be organisms that produce oxygen-dependent metabolites. Some heterofermentative species produce acetic acid, butyric acid (not much in wort, but more so in fermented milk products), and propanoic acid, but they always do so through an anaerobic pathway. The presence of oxygen does not affect metabolism in general [102].
- The major off-flavor that some Lactobacillus strains might produce is acetaldehyde [102]. Saccharomyces will help to metabolize the acetaldehyde produced by Lactobacillus.
Foam Degradation
A few species/strains of Lactobacillus can create all of the amino acids that they need for growth. These species are known as prototrophic. However, most species/strains can only produce some of the amino acids required for growth and must obtain the other amino acids from their environment. These species are known as auxotrophic for the amino acids that they cannot produce themselves [103]. Auxotrophic Lactobacillus can break down proteins in their environment in order to consume the amino acids that they cannot make themselves, including foam forming proteins in beer, through a process called proteolysis [78]. Proteolysis is the breakdown of various proteins into smaller polypeptides or amino acids through the use of various enzymes [104][105][106]. Both homofermentative and heterofermentative species have been observed to have proteolytic activity [107]. This process is a large part of cheese and yogurt fermentation. Lactobacillus species that have been identified as breaking down proteins (mostly in cheese or yogurt) include Lactobacillus bulgaricus, Lactobacillus rhamnosus, Lactobacillus casei, Lactobacillus paracasei, Lactobacillus helveticus, Lactobacillus delbrueckii, Lactobacillus brevis, Lactobacillus cellobiosus, Lactobacillus fermentum, and Lactobacillus plantarum [105]. Results of using various species/strains appears to demonstrate that different species/strains are worse for degrading head retention proteins than others. For example, it's been reported that B.H. Meyer says that souring with L. delbruekii creates better head retention than souring with other species such as L. brevis. [108][109]. Different strains of the same species may also have different levels of proteolytic activity [110].
Proteolytic activity has been shown to decrease as pH falls below 5.0 for some species of Lactobacillus [105]. In order to combat poor head retention in beers that are soured with Lactobacillus, it has been suggested by German brewing scientist, Burghard Hagen Meyer, to lower the pH of the wort to 4.5-4.8 with food grade lactic acid or phosphoric acid before pitching Lactobacillus [111][112][113]. Additionally, ingredients that increase head retention such as unmalted chit, malted wheat, and carafoam have been used to help combat poor head retention in beers soured by Lactobacillus [111][114]. Professional brewer Kristen England of Bent Brewstillery tested Hexa Iso Hop Extract by dosing at 4 times the recommended dosage and found that it greatly increased head retention in a Berliner Weisse (3.5% abv, pH 3.1, TA ~1, BU 5), with a minor taste difference. Kristen recommends experimenting with lower dosages to avoid too much flavor impact.
Another method that has been reported to help with head retention when Sour Worting (kettle souring) is to add a pound of DME per 5 gallons of wort during the heat pasteurization process (after the wort has been soured with Lactobacillus). One could also steep specialty grains such as wheat malt, chit malt, carafoam, or carapils, and add the extract into the kettle during the heat pasteurization or boiling process. This will add back head formation proteins that were lost during the Lactobacillus fermentation [115][116].
See also This discussion with Gareth Young on MTF.
Bacteriocins
While most species of Lactobacillus do not produce bacteriocins, many strains of L. acidophilus are well known for being able to produce bacteriocins, including probiotics and yogurt strains. Bacteriocins are similar to the toxins that some wine yeast strains produce, however bacteriocins tend to target other species that are closely related to the toxin-producing species (although there are some exceptions to this in the literature). The bacteriocin that L. acidophilus produces is a narrow spectrum class II bacteriocin, lactacin B (narrow spectrum means that this toxin kills a very narrow range of closely related Gram-positive bacteria). Species that are susceptible to the lactacin B toxin include species that are genetically closely related to L. acidophilus: L. leichmanii, L. bulgaricus, L. delbruekii, L. lactis, and L. helveticus. Species that are insensitive to the toxin are L. plantarum, L. casei, L. viridescens, and L. fermentum. Some strains of sensitive species may still be insensitive to the toxin from certain strains of L. acidophilus. The toxin does not affect a wide range of bacteria, nor yeast species [117][118].
See Also
Additional Articles on MTF Wiki
- Alternative Bacteria Sources
- Mixed Cultures
- Mixed Fermentation
- Sour Worting
- Scientific Publications
- Pediococcus
- Hops
External Resources
- Microbes.info links to species databases and microbiology resources.
- Physiology of Flavors in Beer – Lactobacillus Species - Matt Humbard's overview of different species of Lactobacillus physiology, discussion on homofermentative vs heterofermentative physiology, which species can ferment different types of sugars, and secondary metabolites. Extensive data points included.
- Beer Microbiology – Lactobacillus pH experiment - Matt Humbard's experiment to determine final pH of individual Lactobacillus strains used in the graphs on this page.
- Lactobacillus Starter Guide by Derek Springer. - Information about starters for both pure strains, as well as culturing from grains.
- Evaluate starter media to propagate Lactobacillus sp., Eureka Brewing Blog, by Samuel Aeschlimann. - This experiment showed that growing Lactobacillus in 10°P DME, 10% apple juice + CaCO3 (20 g L-1) + yeast nutrients lead to the best growth results, and close to expensive MRS media growth results.
- Lacto Starters, by Bryan of Sui Generis blog - Step by step guide to making a starter for Lactobacillus.
- Yeast terminology, part 2: bacteria, by Lars Garshol.
- Elke Arendt, presentation at Belgian Brewing Conference 2015 on scientific findings in LAB in malting and brewing.
- Lactobacillus 2.0 – Advanced Techniques for Fast Souring Beer. Sour Beer Blog.
References
- ↑ 1.0 1.1 1.2 Lactic Acid Bacteria. Todar's Online Texbook of Bacteriology. Kenneth Todar, PhD. Pg. 4. Retrieved 07/28/2015.
- ↑ 2.0 2.1 Nutrition and Growth of Bacteria. Todar's Online Texbook of Bacteriology. Kenneth Todar, PhD. Retrieved 07/28/2015.
- ↑ Lactobacillus. Wikipedia. Retrieved 07/28/2015.
- ↑ "New Culture Pre-Sale July 5: Featuring MTF Mega Blend & Sour Weapon L!" Bootleg Biology website. 06/27/2017. Retreived 06/05/2017.
- ↑ From Gigayeast, Inc. on Facebook, 12/3/2014: "Appears to be L. delbrueckii."
- ↑ Personal Communication with Jim Thompson.
- ↑ 7.0 7.1 7.2 7.3 Lactobacillus 2.0 – Advanced Techniques for Fast Souring Beer. Sour Beer Blog. Matt Miller. 11/18/2015. Retrieved 11/19/2015.
- ↑ GigaYeast Webpage. Retrieved 7/22/2015.
- ↑ Conversation with Steve Smith of GigaYeast on MTF. 05/08/2015.
- ↑ Post on the Lallemand Facebook page. 09/22/2017. Retrieved 09/22/2017.
- ↑ 11.0 11.1 11.2 11.3 11.4 Milk The Funk Wiki. 100% Lactobacillus Fermentation Test by Lance Shaner.
- ↑ 12.0 12.1 12.2 Clarification on optimal OYL-605 temps by Adi hastings on MTF. 06/16/2016.
- ↑ Conversation with Raymond Wagner of Oso Brewing Co on Milk The Funk. 4/30/2015.
- ↑ Conversation with Lance Shaner on MTF in regards to IBU tolerance of OYL-605. 6/15/2015.
- ↑ 15.0 15.1 15.2 Private correspondence with White Labs Customer Service and Dan Pixley. 10/29/2015.
- ↑ Conversation with Nick Impellitteri on MTF regarding TYB Lactobacillus Blend cell counts. 04/08/2016.
- ↑ Impellitteri, Nick. Milk The Funk Facebook group. 03/17/2017.
- ↑ Commercial Brettanomyces, Lactobacillus, and Pediococcus Descriptions. The Mad Fermentationist Blog. Michael Tonsmeire. Retrieved 3/4/2015.
- ↑ Conversation with Andrew Addkison on MTF. 01/12/2016.
- ↑ 20.0 20.1 20.2 20.3 [www.whitelabs.com/sites/default/files/R%26D%20Wild%20Yeast%20and%20Bacteria%20Experiments_2.pdf "R&D Wild Yeast and Bacteria Experiments". White Labs data sheet. Retrieved 05/16/2017.]
- ↑ "Commercial Brettanomyces, Lactobacillus, and Pediococcus Descriptions; Commercial Yeast Laboratories." The Mad Fermentationist blog. Michael Tonsmeire. Retrieved 12/12/2016.
- ↑ 22.0 22.1 Conversation with Nick Impellitteri from The Yeast Bay on the MTF Facebook Group. 3/4/2015.
- ↑ Conversation with Michael Soo and Nick Impellitteri on the Milk The Funk Facebook Group. 3/5/2015.
- ↑ The Yeast Bay website. Retrieved 3/2/2015.
- ↑ 25.0 25.1 Wyeast Specifications 2015 Retail Products. 2015.
- ↑ 26.0 26.1 26.2 26.3 Growth Response of Lactobacillus brevis to Aeration and Organic Catalysts. J. R. Stamer and B. O. Stoyla. Appl Microbiol. Sep 1967; 15(5): 1025–1030.
- ↑ From RVA Yeast Lab's Website
- ↑ Discussion on Milk The Funk Facebook group with Malachy McKenna
- ↑ Conversation with David Thorton on MTF Facebook Group. 2/27/2015.
- ↑ with Lance Shaner and Nick Impellitteri on Lacto starters on MTF. 09/22/2015.
- ↑ Conversation on MTF with Bryan of Sui Generis Blog. 5/6/2015.
- ↑ MTF thread started by Brad Primozic. 5/29/2015.
- ↑ Conversation with Richard Preiss on MTF regarding Lacto starters. 11/19/2015.
- ↑ Conversation with Bryan of Sui Generis Blog on MTF regarding speed of acid production with Lacto. 11/20/2015.
- ↑ 35.0 35.1 "Lacto Starters." Bryan from Sui Generis Blog. Retrieved 6/15/2015.
- ↑ Private correspondence with Richard Preiss to Dan Pixley. 11/20/2015.]
- ↑ The influence of thiamine and riboflavin on various spoilage microorganisms commonly found in beer. Barry Hucker, Melinda Christophersen, Frank Vriesekoop. 2017.
- ↑ Conversation with Bryan of Sui Generis Blog about starters and agitation. 11/09/2015.
- ↑ 39.0 39.1 39.2 Conversation with Sam Aeschlimann of Eureka Brewing Blog on MTF. 08/20/2015.
- ↑ 40.0 40.1 40.2 Examination of Lactobacillus plantarum lactate metabolism side effects in relation to the modulation of aeration parameters. S. Quatravaux, F. Remize, E. Bryckaert, D. Colavizza, J. Guzzo. 2006
- ↑ 41.0 41.1 41.2 Lactobacillus plantarum and its biological implications. Microbe Wiki. Retrieved 6/7/2015.
- ↑ Conversation with Bryan of Sui Generis Blog regarding the use of chalk as a buffer in Lacto starters. 11/20/2015.
- ↑ Conversation with Jeff Mello on MTF regarding using less chalk in LAB starters. 08/10/2016.
- ↑ Impellitteri, Nick. Milk The Funk Facebook group. 03/19/2017.
- ↑ Bryan from Sui Generis. MTF Thread on using 1.5g/L of chalk for Lactobacillus starters. 11/02/2016.
- ↑ 46.0 46.1 Evaluate starter media to propagate Lactobacillus sp., Eureka Brewing Blog, by Samuel Aeschlimann.
- ↑ Conversation with Samuel Aeschlimann and Jason Pappas on MTF about the effects of boiling apple juice. 09/24/2015.
- ↑ Stokes' law. Wikipedia. retrieved 09/24/2015.
- ↑ Conversation with Nick Impellitteri on Milk The Funk Facebook group. 3/5/2015.
- ↑ 50.0 50.1 50.2 50.3 50.4 50.5 50.6 50.7 50.8 50.9 Growth Study, Metabolite Development, and Organoleptic Profile of a Malt-Based Substrate Fermented by Lactic Acid Bacteria. Lorenzo C. Peyer, Emanuele Zannini, Fritz Jacob, and Elke K. Arendt. 2015.
- ↑ Neva Parker, Reddit thread. 10/29/2015.
- ↑ 52.0 52.1 52.2 52.3 Effect of mixed cultures on microbiological development in Berliner Weisse (master thesis). Thomas Hübbe. 2016.
- ↑ Biochemistry. 5th edition. Berg JM, Tymoczko JL, Stryer L. 2002. Chapter 18.
- ↑ Virtual Microbiology Textbook. Department of Bacteriology, University of Wisconsin-Madison. Retrieved 12/02/2015.
- ↑ Bergey's Manual of Systematic Bacteriology, 2nd edition. pg 471
- ↑ Correspondence with Bryan of Sui Generis Blog from Dan Pixley. 12/01/2015.
- ↑ Conversation with Bryan of Sui Generis Blog on MTF regarding butyric acid production by Lactobacillus. 11/23/2015.
- ↑ Physiological role of pyruvate oxidase in the aerobic metabolism of Lactobacillus plantarum. Sedewitz B, Schleifer KH, Götz F.1984.
- ↑ 59.0 59.1 Conversation with Lance Shaner about L. plantarum on MTF. 6/7/2015.
- ↑ The oxygen-inducible conversion of lactate to acetate in heterofermentative Lactobacillus brevis ATCC367. Tingting Guo, Li Zhang, Yongping Xin, ZhenShang Xu, Huiying He, and Jian Kong. 2017.
- ↑ MTF conversation with Adi Hastings from Omega Yeast Labs on the effects of oxygen presence in OYL-605. 02/02/2016.
- ↑ Conversation 3 with Bryan of Sui Generis Blog on Milk The Funk regarding Lactobacillus hop tolerance. 09/30/2016.
- ↑ Conversation 1 with Bryan of Sui Generis Blog on Milk The Funk regarding Lactobacillus hop tolerance. 01/19/2015.
- ↑ Conversation 2 with Bryan of Sui Generis Blog on Milk The Funk regarding Lactobacillus hop tolerance. 09/28/2015.
- ↑ 65.0 65.1 Methner, F.D. Uber Die Aromabildung beim berliner weissebier unter besonderer berucksichtigung von sauren and estern (data reported and translated by Benedikt Rausch on Milk THe Funk Facebook group). 1987.
- ↑ Ethanol tolerance and carbohydrate metabolism in lactobacilli. R. Shane GoldM. M. MeagherR. HutkinsT. Conway. 1992.
- ↑ 67.0 67.1 67.2 67.3 67.4 Sour Brewing: Impact of Lactobacillus amylovorus FST2.11 on Technological and Quality Attributes of Acid Beers. Lorenzo C. Peyer, Martin Zarnkow, Fritz Jacob, David P. Schutter, Elke K. Arendt. 2017.
- ↑ Fermentation nation Podcast interview with Jessica Davis, QA for The Bruery.
- ↑ Conversation with Bryan of Sui Generis Blog on stress factors in storing Lacto. 09/28/2015.
- ↑ Conversation with Bryan of Sui Generis Blog on Milk The Funk. 05/04/2015.
- ↑ Conversation with Adi Hastings on MTF. 6/20/2015.
- ↑ Conversation with Tom Belgrano on MTF regarding storing Lacto. 09/28/2015.
- ↑ Conversation with Bryan of Sui Generis blog on storing Lactobacillus in wort. 11/02/2016.
- ↑ Conversation with Logan Blancett regarding tyndallization and botulism toxin. 11/02/2016.
- ↑ Sharing Dr. Roy Ventullo's experiment results comparing Samuel Aeschlimann's "Eureka" Lactobacillus starter versus traditional MRS media on MTF. 09/23/2016.
- ↑ Rogosa SL Agar (Lactobacillus). Basement Brew Lab. Dustin Metzger. Retrieved 12/23/2015.
- ↑ 77.0 77.1 77.2 77.3 77.4 77.5 Fermentation: Effects on Food Properties. Bhavbhuti M. Mehta, Afaf Kamal-Eldin, Robert Z. Iwanski. CRC Press, Apr 12, 2012. Pg 76,77.
- ↑ 78.0 78.1 Todar's Online Texbook of Bacteriology. Kenneth Todar, PhD. Retrieved 05/06/2015.
- ↑ Lactic Acid Bacteria. Raunak Shrestha. Retrieved 6/7/2015.
- ↑ Handbook of Dough Fermentations. Karel Kulp, Klaus Lorenz. CRC Press, May 20, 2003. Pg 33.
- ↑ Understanding Bacteria. S. Srivastava. 2013. Pg 157.
- ↑ Todar's Online Textbook of Bacteriology. Diversity of Metabolism in Procaryotes (page 3). Retrieved 12/29/2015.
- ↑ Microbiology. Daniel V. Lim. 2003. Pg 180.
- ↑ Lactic Acid Bacteria: Microbiological and Functional Aspects, Fourth Edition. Sampo Lahtinen, Arthur C. Ouwehand, Seppo Salminen, Atte von Wright. CRC Press, Dec 13, 2011. Pg 80.
- ↑ Complete Genome Sequence of the Probiotic Lactic Acid Bacterium Lactobacillus Rhamnosus. Samat Kozhakhmetov, Almagul Kushugulova, Adil Supiyev, Indira Tynybayeva, Ulykbek Kairov, Saule Saduakhasova, Gulnara Shakhabayeva, Kenzhebulat Bapishev, Talgat Nurgozhin, Zhaxybay Zhumadilov. 2013.
- ↑ 86.00 86.01 86.02 86.03 86.04 86.05 86.06 86.07 86.08 86.09 86.10 86.11 86.12 86.13 86.14 86.15 86.16 86.17 86.18 Fermented Fruits and Vegetables. A Global Perspective. Food and Agriculture Organization of the United Nations. Chapter 5, Bacterial Fermentations. Retrieved 11/15/2015.
- ↑ 87.0 87.1 87.2 87.3 87.4 87.5 87.6 Lactic Acid Bacteria as Sensory Biomodulators for Fermented Cereal-Based Beverages. Lorenzo C. Peyer , Emanuele Zannini , Elke K. Arendt. 2016.
- ↑ Screening of Lactobacillus spp. and Pediococcus spp. for glycosidase activities that are important in oenology. A. Grimaldi, E. Bartowsky, V. Jiranek. 2005. DOI: 10.1111/j.1365-2672.2005.02707.x.
- ↑ Conversation with Richard Preiss on MTF regarding pure Lactobacillus fermentation. 07/19/2016.
- ↑ Discussion with Lance Shaner on MTF. 6/7/2015.
- ↑ Humbard, Matt. Physiology of Flavors in Beer – Lactobacillus Species. Retrieved 6/14/2015.
- ↑ 92.0 92.1 Key volatile aroma compounds of lactic acid fermented malt based beverages – impact of lactic acid bacteria strains. Sorelle Nsogning Dongmo, Bertram Sacher, Hubert Kollmannsberger, Thomas Becker. 2017. doi:http://dx.doi.org/10.1016/j.foodchem.2017.02.091.
- ↑ The natural functions of secondary metabolites. Demain AL, Fang A. 2000.
- ↑ Private correspondence with Richard Preiss from Dan Pixley. 12/29/2015.
- ↑ Leffingwell & Associates website. Odor Thresholds. Retrieved 12/30/2015.
- ↑ Mousy Off-Flavor of Wine: Precursors and Biosynthesis of the Causative N-Heterocycles 2-Ethyltetrahydropyridine, 2-Acetyltetrahydropyridine, and 2-Acetyl-1-pyrroline by Lactobacillus hilgardii DSM 20176. Peter J. Costello and Paul A. Henschke. 2002.
- ↑ Volatile compounds produced by the probiotic strain Lactobacillus plantarum NCIMB 8826 in cereal-based substrates Ivan Salmeron, Pablo Fuciños, Dimitris Charalampopoulos, Severino S. Pandiella. 2009.
- ↑ Purification and Characteristics of Tannase Produced by Lactic Acid Bacteria, Lactobacillus plantarum H78. Mari Matsuda, Yayoi Hirose, and Makoto Kanauchi. 2016.
- ↑ "http://www.beveragedaily.com/R-D/New-enzyme-aims-to-take-the-haze-out-of-iced-tea". Beveragedaily.com. Guy Montague-James. 04/04/2011. Retrieved 011/09/2016.
- ↑ Review of this entry by Mike Lentz via MTF. 11/10/2016.
- ↑ Secondary Metabolite. Wikipedia. Retrieved 6/9/2015.
- ↑ 102.0 102.1 Conversation with Bryan of Sui Generis Blog on the effects of oxygen in LAB on MTF. 11/23/2015.
- ↑ Phenotypic and genotypic analysis of amino acid auxotrophy in Lactobacillus helveticus CNRZ 32. Christiansen JK, Hughes JE, Welker DL, Rodríguez BT, Steele JL, Broadbent JR. 2007.
- ↑ Texture, proteolysis and viable lactic acid bacteria in commercial Cheddar cheeses treated with high pressure. Cheryl Wick, Uwe Nienaber, Olga Anggraeni, Thomas H Shellhammer and Polly D Courtney. 2002. Retrieved 7/7/2015.
- ↑ 105.0 105.1 105.2 Protease Biosynthesis from Lactobacillus Species: Fermentation Parameters and Kinetics. Ikram-Ul_Haq and Hamid Mukhtar. Jan 2007. Retrieved 7/7/2015.
- ↑ Proteolysis. Wikipedia. Retrieved 7/7/2015.
- ↑ Brewing Microbiology. Fergus Priest. Springer Science & Business Media, Jun 29, 2013. Pg 133.
- ↑ Conversation with Kristen England on MTF. 7/7/2015.
- ↑ Conversation with Jace Marti on MTF about L. delbruekii head retention problems. 6/21/2015.
- ↑ Comparison of proteolytic activities in various lactobacilli. Sasaki M, Bosman BW, Tan PS. 1995.
- ↑ 111.0 111.1 Berliner Weisse – the old-time kettle-souring technique. Brewing Sour blog, by Gail Ann Williams.September 18, 2012. Retrieved 7/7/2015.
- ↑ Berliner Weisse Test. Ingenuity Brew Blog. June 4, 2013. Retrieved 7/7/2015.
- ↑ 100% Lactobacillus Berliner Weisse. The Mad Fermentationist Blog, by Michael Tonsmeire. June 25, 2012. Retrieved 7/7/2015.
- ↑ Conversation with Richard Preiss on MTF. 7/7/2015.
- ↑ Conversation with Gareth Young on MTF. 05/08/2015.
- ↑ Conversation with Paul Finney on MTF in regards to head retention of Berliner Weisse. 08/29/2015.
- ↑ Detection and activity of lactacin B, a bacteriocin produced by Lactobacillus acidophilus. S F Barefoot and T R Klaenhammer. 1983.
- ↑ Lack of Heterogeneity in Bacteriocin Production Across a Selection of Commercial Probiotic Products. J. W. Hegarty, C. M. Guinane, R. P. RossC. Hill, P. D. Cotter. 2017.